Eco-Innovations in Energy Production: Technologies to Reduce Lubricant Consumption
Introduction
Power generation facilities rely on an array of lubricants to ensure the dependable production of electricity. The critical nature of these lubricants cannot be overstated; for instance, the operation of a large steam turbine, which may have a shaft weighing dozens of tons and rotating at speeds of 3,000 revolutions per minute, depends on a 20-micron oil film. This critical barrier is all that separates the turbine from reliable energy production from catastrophic failure. There is a growing pressure on energy production facilities to reduce their environmental impact by diminishing waste and lowering their carbon footprint. This environmental focus has now extended to lubricant management, with a significant shift in perspective from viewing lubricants as mere consumables to treating them as valuable assets. Consequently, many power plants have adopted condition-based maintenance strategies for lubricants, moving away from the traditional schedule-based replacements. The simultaneous goals of reducing lubricant consumption and enhancing plant availability can be aligned. This paper aims to explore how the adoption of cutting-edge technologies can achieve both reduced lubricant use and increased operational efficiency in power plants.
The Environmental Impact of Extending Lubricant Lifespan
A comprehensive Life Cycle Assessment (LCA) reveals that the significant carbon footprint of lubricants predominantly accrues at the end of their lifecycle, with incineration emitting double the carbon dioxide compared to the combined processes of extraction, refinement, additive blending, and packaging (see Fig. 1).
Figure 1: Life Cycle Assessment of a lubricant reveals that incinerating oil at the end of its life consumes twice as much carbon as the entire extraction, refining, blending and packaging stages of a lubricant’s life.
Doubling the life of a lubricant therefore will allow you to cut your carbon footprint in half.
“Product Use” is Paramount
Merely prolonging the intervals between lubricant changes can lead to adverse outcomes if improperly managed. The “Product Use” phase is identified as the most critical in terms of carbon footprint impact within the LCA framework. Irresponsibly extending lubricant life might lead to equipment failure, potentially resulting in a greater carbon footprint than the cumulative use of lubricants. Lubricants are also essential for reducing energy consumption and enhancing machinery efficiency through friction reduction, wear and tear minimization, and improved heat dissipation.
Prioritizing Lubricant Performance
The first objective in any lubricant consumption strategy should be to optimize lubricant performance. Life-extension strategies should be pursued only when they do not compromise lubricant efficacy. Technological advancements have made it possible to achieve both enhanced lubricant performance and extended life.
Implementing an effective lubrication program is a multidisciplinary effort that demands organizational support and encompasses lubricant selection, handling, storage, contamination control, analysis, and training. The “ICML 55.2 Standard: Guideline for the Optimized Lubrication of Mechanical Physical Assets” serves as an excellent resource for developing a top-tier lubrication program.
This paper will further explore the largest volume application in power plants and investigate new technologies aimed at optimizing both performance and lifespan.
Turbine Oils
Turbine oil, the highest-volume lubricant in power plants, presents a prime opportunity for reducing lubricant consumption. Its application extends beyond turbines to feed pumps and steam loops.
Selection Criteria
Not all turbine oils offer the same performance despite meeting Original Equipment Manufacturer (OEM) specifications. The purchasing decisions often hinge on cost and compliance with OEM specifications, overlooking the total cost of ownership (TCO). Given turbine oil’s significant volume and critical role in power plant operations, the choice of oil—ideally by the Engineering Department over the Purchasing Department—has profound implications. Our benchmark evaluations of commercially available turbine oils have revealed marked differences in their degradation rates, lifespan, and resistance to varnish and deposit formation, as can be seen in Fig. 2.
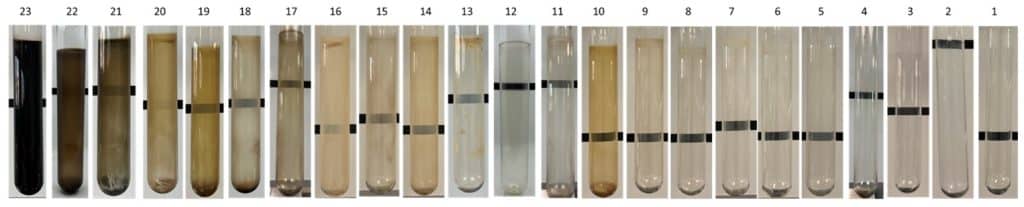
Figure 2: An example of glassware in an accelerated aging test comparing 23 commercially available turbine oils illustrating significant differences in deposit performance of various formulations.
Base Oil Consideration
Much attention has been given to the transition from API Group I base oils in turbine oil formulations to API Group II or III base oils as the cause for varnish. This is because Group I’s have a higher solubility and are thought to hold more deposits compared to Group II or IIIs. This characteristic can be estimated by the base oil’s aniline point. The components in Group I base oils that allow them to have greater solubility are sulfur and aromatic constituents. Ironically, these impurities also decrease the oxidative stability of a turbine oil formulation and contribute to more varnish. None of the turbine oils tested in Fig. 2 are Group I formulations as they are unable to survive the 12-week aging test and produce extremely high levels of deposits. Unless you are using very old turbine technology, you should only consider a Group II or higher formulation for long-life and low deposits.
Condition Monitoring
Turbine oil degradation primarily stems from oxidation, leading to antioxidant depletion and deposit formation. Essential for monitoring turbine oil life are oxidation stability and varnish potential, assessed via RPVOT (ASTM D2272), RULER (ASTM D6971), and MPC (ASTM D7843) tests. Excluding these analyses could obscure emerging problems. A comprehensive analysis program also includes tests for viscosity, acid number, elemental content, particle count, water content, demulsibility, air release, foam tendency, and rust prevention.
Inline AnalysisRecent advancements in sensor technology now enable real-time turbine oil analysis. Mid-infrared sensors can measure antioxidants, degradation products, and water content, offering immediate insights into oil condition. An example of this technology can be seen in Fig. 3.
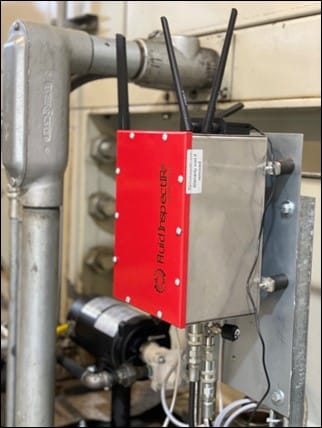
Figure 3: Example of a FluidInspecIR sensor system providing real time turbine oil monitoring in a gas turbine.
Achieving Varnish-Free Operation
The primary indicator of turbine oil failure is deposit formation. Turbine oil varnish is the issue that threatens reliable energy production more than any other lubricant related problem. In addition, varnish creates a need for chemical flushing to decontaminate lube oil systems in between oil changes, which not only consumes significant volumes of oil but is also costly. However, it is possible to achieve varnish-free turbine operations. The two leading technologies to accomplish this are resin-based kidney loop filtration systems and solvency enhancers. Both technologies have been shown to provide long-term freedom from varnish. However, care should be taken when using solvency enhancers, as they are not all designed for long-term use, as can be seen in Fig. 4.
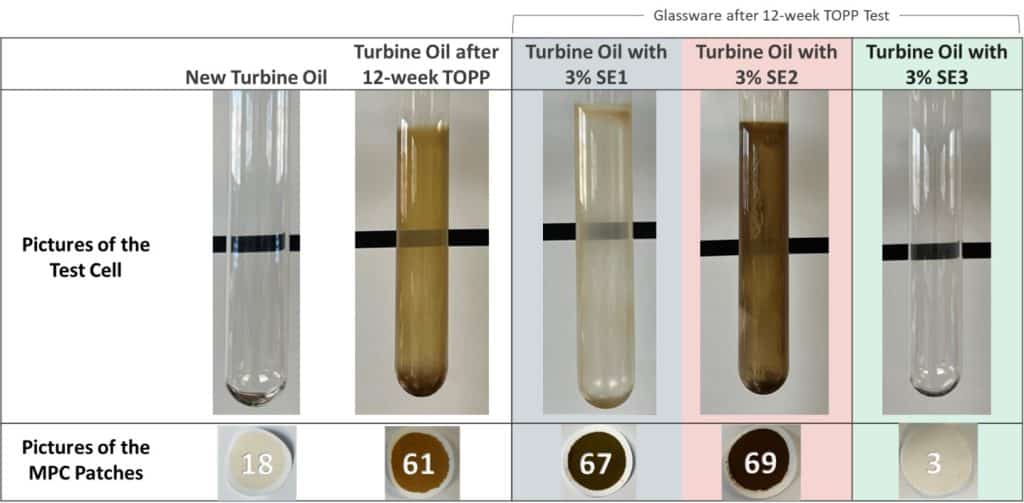
Figure 4: Benchmarking tests from 3 different turbine oil solvency enhancers, showing the differences in long-term performance between different technologies. Not all solvency enhancers are designed for long-term use.
Maintaining Antioxidant Health
Antioxidants are crucial in preventing oil degradation. Once depleted, the base oil has no protective capabilities against oxidation resulting in rapid failure, as shown in Fig. 5. Not only was this turbine oil completely devoid of antioxidants, but a coolant leak entered the system causing rapid fluid degradation and turbine failure. Ironically, the plant did monthly oil analysis, however, did not measure antioxidant health or varnish potential. Only in extreme cases are viscosity and acid number useful indicators of turbine oil health or lack thereof.
Figure 5: Rapid, catastrophic failure of a turbine due to a simultaneous event of the oil running in the absence of antioxidants and a coolant leak. Once can observe the viscosity increase to 100 cSt @40C over the course of a month and the acid number increasing to almost 20.
When antioxidants fall below 50% of their new oil value, proactive action should be considered. This provides sufficient time prior to the oil reaching its 25% condemning limit. Often a new oil purchase is considered at this stage. However, if the plant is wishing to both reduce oil consumption while optimizing performance, online antioxidant addition is also a possibility to restore antioxidant health. Before adding any new chemistry to an in-service turbine oil, compatibility testing should be done to identify any potential adverse reactions. The same goes for in situ antioxidant replenishment. If up-front simulation testing is done, antioxidant replenishment has been shown to be a safe, effective way of doubling turbine oil life. An example of this can be seen in Fig. 6 where a turbine oil’s life has been extending from 8 years to an estimated 30 years in a GE Frame 7 large frame gas turbine.
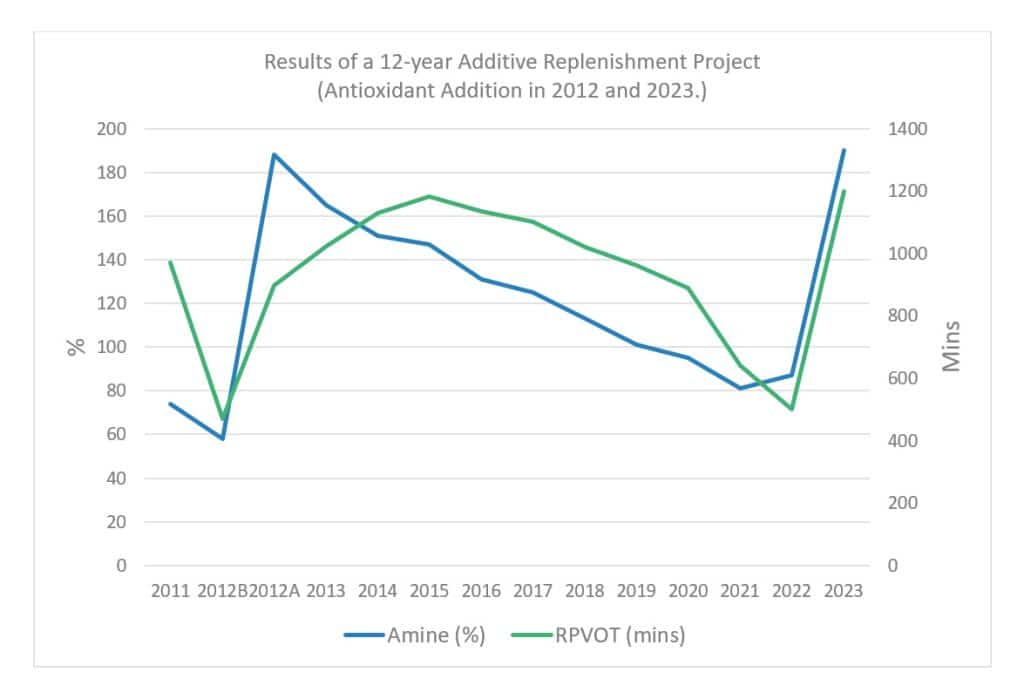
Figure 6: An example of online additive replenishment performed in 2012 and then again in 2023 in a large frame gas turbine. In addition to the RPVOT and amine antioxidants being restored, the MPC levels are maintained to <10. (As of this writing, the MPC was 2.) Estimated life of this oil is expected to be >30 years based on it’s current rate of degradation.
Balance of Plant Lube Oil Systems
Although the focus of this paper has been on the highest volume lubricant at a power plant, there are multiple other applications where both oil life and performance can be optimized, including the following:
Gearbox systems
Cooling towers are an extremely stressful environment for lubricants as they operate in very hot, humid environments. Upgrading cooling tower gearbox oil from a mineral oil formulation to a fully synthetic, polyalphaolefin (PAO) based formulation will significantly increase the life of the oil, reduce deposits and oxidation, and lead to increased energy efficiency.
Coal pulverizer gearboxes may also benefit from an upgrade to a synthetic PAO formulation, however the biggest issue in these applications is coal-dust contamination. A kidney loop capillary filtration system will significantly improve gearbox life and oil performance.
Steam Turbine Electrohydraulic Control (EHC) Systems
Most OEMs require the use of phosphate ester fluids in EHC systems due to their self-extinguishing properties, making them the safest fluid choice. Although phosphate esters used in EHC systems are typically additive-free, they can be complex to maintain. Hydrolysis and oxidation are their primary modes of degradation, so they require appropriate technologies to control this and provide long fluid life. The leading contamination control technologies are ion exchange acid scavenging filters and dry-air blanketing systems to control moisture levels. One other type of degradation that may occur in some EHC systems is micro-dieseling, resulting in the formation of submicron carbonaceous particles. Although there are multiple actions that can be taken to fix the root cause of micro-dieseling, a permanent band-aid may be the use of a kidney loop filter using either capillary or electrostatic technology. Ultimately, well maintained EHC fluids can last for decades, however further details about phosphate ester management are beyond the scope of this article.
Conclusion
Significant volumes of lubricant are required in the generation of energy. There are multiple strategies that can be employed to optimize the life and performance of these critical lubricants.
Lubricants are pivotal for the reliable operation of power generation equipment, with their management evolving from consumable assets to valuable components that require strategic conservation.
- Lubricants are pivotal for the reliable operation of power generation equipment, with their management evolving from consumable assets to valuable components that require strategic conservation.
- Extending the lifespan of lubricants significantly reduces their environmental impact, particularly in reducing carbon emissions during the disposal phase.
- Optimization of lubricant performance should precede life-extension strategies to ensure equipment reliability and efficiency.
- Technological advancements have enabled more effective monitoring and maintenance of lubricants, contributing to both extended lubricant life and enhanced operational performance.
- Turbine oils, as the highest-volume lubricant in power plants, offer substantial opportunities for consumption reduction through careful selection, condition monitoring, and varnish-free operation.
- Innovative maintenance technologies, such as resin-based filters and solvency enhancers, have proven effective in extending oil life and preventing common issues like varnish and deposit formation.
- Proactive antioxidant management including additive replenishment can double or even quadruple the life of turbine oil, significantly impacting both environmental sustainability and operational cost-effectiveness.
- Beyond turbines, other plant systems like gearbox and EHC systems also benefit from advanced lubricant management strategies, highlighting the importance of a comprehensive approach to lubrication in power plants.
The integration of advanced technologies and strategic management practices in lubrication processes not only enhances the efficiency and reliability of power generation facilities but also contributes significantly to environmental conservation efforts
Author